Elastic anisotropy of experimental analogues of perovskite and post perovskite help to interpret D” diversity
March 27, 2014
Okayama University
University of Hyogo
RIKEN
Japan Synchrotron Radiation Research Institute
University of Hyogo
RIKEN
Japan Synchrotron Radiation Research Institute
Nature Communications 5, Article number: 3453, doi:10.1038/ncomms4453 (March 27, 2014)
Authors: Akira Yoneda1, Hiroshi Fukui2,3, Fang Xu1, Akihiko Nakatsuka4, Akira Yoshiasa5, Yusuke Seto6, Kenya Ono7, Satoshi Tsutsui8, Hiroshi Uchiyama8 & Alfred Q. R. Baron3,8
Corresponding author: Akira Yoneda
1Institute for Study of the Earth’s Interior, Okayama University, Misasa, Tottori 682-0193, Japan.
2Graduate School of Material Science, University of Hyogo, Kamigori, Hyogo 678-1297, Japan.
3Materials Dynamics Laboratory, RIKEN SPring-8 Center, RIKEN, Hyogo 679-5148, Japan.
4Graduate School of Science and Engineering, Yamaguchi University, Ube, Yamaguchi 755-8611, Japan.
5Graduate School of Science and Technology, Kumamoto University, Kumamoto 860-8555, Japan.
6 Graduate School of Science, Kobe University, Kobe 657-8501, Japan.
7Department of Earth Sciences, University of Toyama, Toyama 930-8555, Japan.
8Japan Synchrotron Radiation Research Institute, Sayo, Hyogo 679-5198, Japan.
Dr. Akira Yoneda (Okayama Univ.), Dr. Hiroshi Fukui(Univ. of Hyogo), Dr. Alfred Q. R. Baron (RIKEN), and Dr. Satoshi Tsutsui (JASRI) measured single crystal elasticity of perovskite Pbnm-CaIrO3 and post perovskite Cmcm-CaIrO3 by using BL35XU, SPring-8. Those materials are analogues of perovskite and post perovskite MgSiO3. The results were reported on Nature Communications;
Nature Communications 5, Article number: 3453, doi:10.1038/ncomms4453 (March 27, 2014)
Recent studies show that the D" layer, just above the Earth's core-mantle boundary, is composed of MgSiO3 post perovskite and has significant lateral inhomogeneity. Here we consider the D" diversity as related to the single crystal elasticity of the post perovskite phase. We measure the single-crystal elasticity of the perovskite Pbnm-CaIrO3 and post perovskite Cmcm-CaIrO3 using inelastic X-ray scattering. These materials are structural analogues to same phases of MgSiO3. Our results show that Cmcm-CaIrO3 is much more elastically anisotropic than Pbnm-CaIrO3, which gives an explanation for enigmatic seismic wave velocity jump at the D’’ discontinuity. Considering a relation between lattice preferred orientation and seismic anisotropy in the D" layer, we suggest that the c axis of post perovskite MgSiO3 aligns vertically beneath the Circum-Pacific rim, and the b axis vertically beneath the Central Pacific.
This study was supported by Grants-in-Aid for Scientific Research from Japan Society for the Promotion of Science (JSPS):
Scientific Research (S) “Experimental study on physical properties of minerals at the lowermost mantle conditions by means of Kawai-type apparatus” to Akira Yoneda.
<Background>Nature Communications 5, Article number: 3453, doi:10.1038/ncomms4453 (March 27, 2014)
Recent studies show that the D" layer, just above the Earth's core-mantle boundary, is composed of MgSiO3 post perovskite and has significant lateral inhomogeneity. Here we consider the D" diversity as related to the single crystal elasticity of the post perovskite phase. We measure the single-crystal elasticity of the perovskite Pbnm-CaIrO3 and post perovskite Cmcm-CaIrO3 using inelastic X-ray scattering. These materials are structural analogues to same phases of MgSiO3. Our results show that Cmcm-CaIrO3 is much more elastically anisotropic than Pbnm-CaIrO3, which gives an explanation for enigmatic seismic wave velocity jump at the D’’ discontinuity. Considering a relation between lattice preferred orientation and seismic anisotropy in the D" layer, we suggest that the c axis of post perovskite MgSiO3 aligns vertically beneath the Circum-Pacific rim, and the b axis vertically beneath the Central Pacific.
This study was supported by Grants-in-Aid for Scientific Research from Japan Society for the Promotion of Science (JSPS):
Scientific Research (S) “Experimental study on physical properties of minerals at the lowermost mantle conditions by means of Kawai-type apparatus” to Akira Yoneda.
Since the discovery of the transition from perovskite (Pv) to post perovskite (pPv) in MgSiO3, pPv-MgSiO3 has been considered to be a major component of the D" layer just above the core–mantle boundary. Seismological observations of the D" layer are difficult to interpret as they suggest both non-uniform response at the layer boundary and non-uniform anisotropy inside the layer. With this background, elastic anisotropy, or single-crystal elasticity, of pPv-MgSiO3 has been the focus of theoretical calculations. However, no experimental data are available on the single-crystal elasticity of pPv-MgSiO3, because it is unstable at ambient pressure.
Cmcm-CaIrO3, or pPv-CaIrO3, has been frequently selected as a representative analog of pPv-MgSiO3. According to the phase diagram, pPv-CaIrO3 is the stable phase at ambient conditions and Pv-CaIrO3 is the high-temperature and low-pressure phase. Here we report the first experimental data on single crystal elasticity of Pv and pPv structures in a homogeneous chemical composition of CaIrO3.
Figure 1 shows single crystals of Pv-CaIrO3 and pPv-CaIrO3 used in the present study. The Pv-CaIrO3 sample was synthesized at 2 GPa and 1450℃ for 15 h in a piston cylinder apparatus at Okayama University. We produced many grains with size of a few tens of microns, although most of them turned out to have multiple domains. We examined more than 20 grains using a four-circle X-ray diffractometer at Yamaguchi University and selected a quasi-single domain crystal with size of ~20 μm. Its lattice constants were determined as a = 5.3527(1), b = 5.5969(5), and c =7.6804(6) Å, which yields density (p) of 8091 kg/m3. The pPv-CaIrO3 sample was synthesized by slow cooling from 1000℃ in CaCl2 flux15. It had a pine-needle shape with length of a few hundred microns and width of a few tens of microns. Its lattice constant and densities were respectively a = 3.145(2), b = 9.861(6), and c = 7.297(5) Å, and p= 8211 kg/m3, which are consistent with literature values.
<Method and results>
Measurement of IXS at SPring-8*1 BL35XU. The small size and black color of the present samples limit the techniques that can be used to investigate the elastic properties. In particular, the crystal sizes are too small to employ GHz ultrasonic methods or resonant ultrasound spectroscopy, which both need crystals larger than 100 μm. In addition, the crystal opacity makes it difficult to apply Brillouin scattering. Therefore, IXS with an X-ray beam diameter of ~70 μm was employed. Each specimen was attached to glass fibers (~100 μm in diameter) using manicure resin and mounted on the four-circle goniometer installed at BL35XU of SPring-8. The incident X-ray beam energy was 17.794 and 21.747 keV for Pbnm-CaIrO3 and Cmcm-CaIrO3 measurements, respectively.
The IXS technique allows us to determine the elastic properties of a material by measuring the energies of acoustic longitudinal and shear phonon modes. When this information is combined with the precise reciprocal space position (momentum transfer) for each measurement, we can determine the sound velocity. If measurements are made in many different directions, the velocity information can be combined with the density, allowing all the elastic constants of a material to be determined. In the present work, the two-dimensional, 4 × 3, analyzer array of BL35XU is especially advantageous as it allows access to 12 different directions of momentum transfer simultaneously.
Figure 3 summarizes the present idea for seismic anisotropy and LPO structure in the D" layer. The VTI of the vertical c axis and that of the vertical b axis can be interpreted as transformation LPO and deformation LPO, respectively. It is noted that isotropic pPv-MgSiO3 is a reasonable interpretation for the seismic feature beneath the Atlantic Ocean; an alternative interpretation may be the VTI of the vertical a axis because of less lateral polarization anisotropy than in other cases. The difference between beneath the Pacific and Atlantic may be attributed to differences in temperature, chemical composition, and strain rate. The proposed LPO behavior for pPv-MgSiO3 obtained from the present mineral physics perspective is consistent with seismic observation.
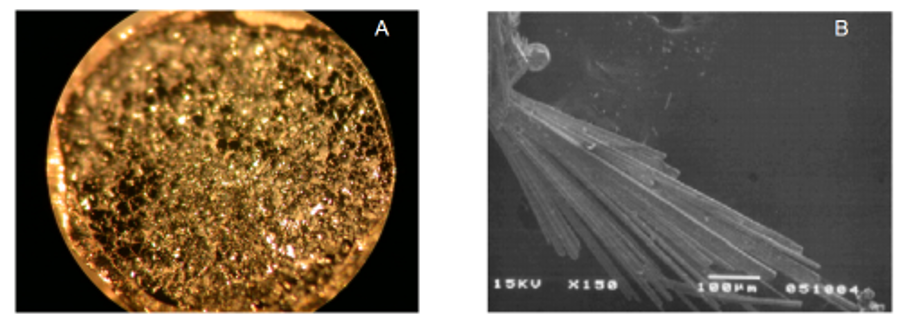
Figure 1 Photograph of single crystals used. A and B refer to Pbnm- and Cmcm-CaIrO3, respectively. The horizontal edge lengths are ~5 mm and ~800 μm, respectively, for A and B.
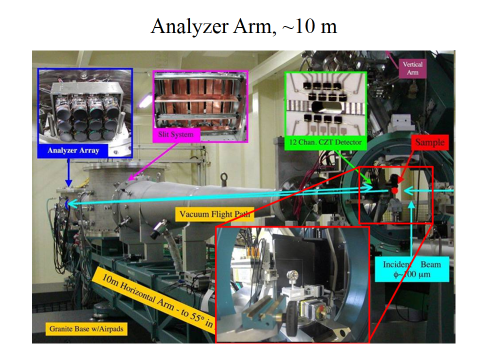
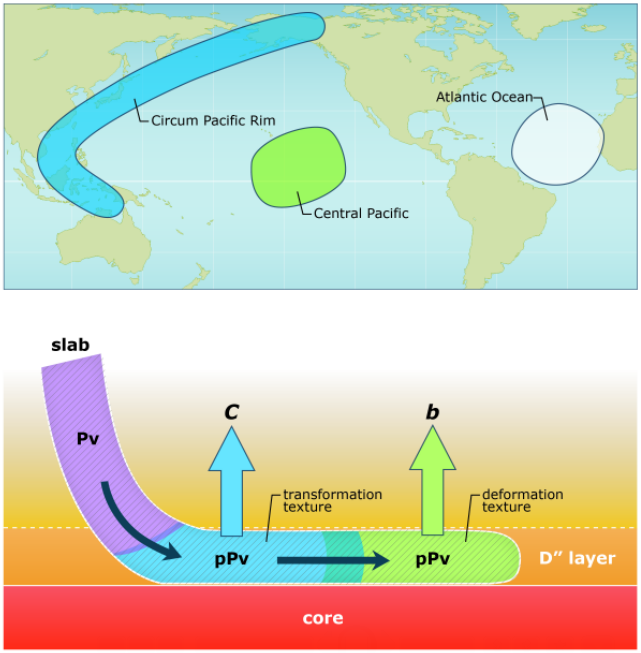
Figure 3 Schematic illustration of the LPO of pPv-MgSiO3 in the D" layer. From a mineral physics viewpoint, the Pv slab (pink region) transforms to pPv (light blue region) and the pPv slab then moves along the path of mantle convection with deformation (light-green region). In contrast, from seismic observation, the region of the Circum-Pacific rim has VTI anisotropy of VSH > VSV, while the region of the Central Pacific has complicated VTI with any magnitude relation; i.e., VSH > VSV, VSH < VSV or VSH ≈ VSV. The region of the Atlantic Ocean (white circle) has VTI with VSH ≈ VSV, suggesting isotropy or negligible LPO of pPv MgSiO3. The speculated aligned axes of pPv LPO are shown by arrows for each region; the vertical c axis beneath the Circum Pacific Rim and the vertical b axis beneath the Central Pacific.
*1 SPring-8
SPring-8 is a synchrotron radiation facility that provides the world’s highest-brilliance synchrotron radiation. It is owned by RIKEN and located in Harima Science Park City, Hyogo Prefecture, Japan. JASRI is responsible for the operation, management, and promotion of the use of SPring-8. The name “SPring-8” is derived from “Super Photon ring-8 GeV”. When the direction of the electron beams accelerated to nearly the speed of light is changed by magnets, electromagnetic waves are emitted in the tangential direction; these waves are synchrotron radiation. When the electron beam has a higher energy and the change in the traveling direction is large, synchrotron radiation contains shorter-wavelength lights such as X-rays. In particular, the following three facilities are known as the third-generation large synchrotron facilities: SPring-8 in Japan, the Advanced Photon Source (APS) in the USA, and the European Synchrotron Radiation Facility (ESRF) in France. Because the ring at SPring-8 enables an electron acceleration energy of 8 giga-electronvolts to be generated, synchrotron radiation in a wide range of wavelengths can be obtained including far-infrared light, visible light, vacuum ultraviolet light, soft X-rays, and hard X-rays. SPring-8 is used by researchers both in Japan and overseas for joint research in various fields such as materials science, earth science, life science, environmental science, and industrial applications.
<Inquiry>
Institute for Study of the Earth's Interior Okayama University
Akira Yoneda
TEL:+81-858-43-3762
FAX:+81-858-43-2184
homepage:http://www.misasa.okayama-u.ac.jp/~hacto/top_e.html