- Home »
- Research »
- Projects
Our Research Projects
The human body is made up of more than 200 different types of cells. The ultimate purpose of our research is to examine the etiology of human diseases and to find a cure for them. As a first step in achieving this goal, we are conducting basic biomedical studies to identify cellular types from various organs and tissues and clarifying the molecular and cellular mechanisms of functional cytodifferentiation. We are also interested in basic and applied sciences regarding regeneration and remodeling of tissue and organs.
1. Studies on functions and mechanisms of differentiation of novel opsin (photopigment)-expressing cells in the retina, brain, and endocrine organs
The vertebrate retina consists of morphologically and functionally different cells; therefore, it is a paradigm to study the mechanisms of cellular differentiation. Previous studies have shown that more than 50 retinal subtypes are found in one retinal cell type, for example, the retinal ganglion cell, which is a retinal neuron that projects to the brain. However, the mechanism for the emergence of these numerous retinal subtypes and their functions remains unclear. Our recent studies have shown that novel opsin (a protein moiety of a photo-pigment) genes are expressed in small subsets of retinal and brain cells, which are not classical photoreceptor cells. For example, opsin 5 is identified as an ultraviolet sensor (see refs). This indicates that novel types of photoreceptors are present in the retina and brain, and it is intriguing to know the functions of such potential photosensitive cells. Do these cells actually detect light of short wavelengths and for what? Also, we aim to clarify the regulatory mechanisms of such photosensitive gene expression and to study the basic mechanisms of cellular differentiation of the retina and other organs. [The figure shows opsin 5-immunoreactive cells (black signals) in the chick hypothalamus, the paraventricular organ, which is photosensitive in non-mammalian vertebrates such as birds and reptiles (see ref. 2)].
Recently, we started applying genome-editing technique to model organism medaka and analyzing the morphology, behavior, and gene expression profiles of genome-edited medaka fishes to reveal the physiological function and the regulation of gene expression of opsin 5.
References:
- Evolution of Mammalian Opn5 as a Specialized UV-absorbing Pigment by a Single Amino Acid Mutation. Yamashita T, Ono K, Ohuchi H, Yumoto A, Gotoh H, Tomonari S, Sakai K, Fujita H, Imamoto Y, Noji S, Nakamura K, Shichida Y. J Biol Chem. 2014 Feb 14;289(7):3991-4000. doi: 10.1074/jbc.M113.
- A non-mammalian type opsin 5 functions dually in the photoreceptive and non-photoreceptive organs of birds. Ohuchi H, Yamashita T, Tomonari S, Fujita-Yanagibayashi S, Sakai K, Noji S, Shichida Y. PLoS One. 2012;7(2):e31534.
- Opn5 is a UV-sensitive bistable pigment that couples with Gi subtype of G protein. Yamashita T, Ohuchi H, Tomonari S, Ikeda K, Sakai K, Shichida Y. Proc Natl Acad Sci U S A. 2010 Dec 21;107(51):22084-9.
- Expression patterns of the opsin 5-related genes in the developing chicken retina. Tomonari S, Migita K, Takagi A, Noji S, Ohuchi H. Dev Dyn. 2008 Jul;237(7):1910-22.
2. Studies on pattering and morphogenesis of the optic vesicle (eye primordium)
The retina consists of the neural retina (NR), which contains various types of retinal neurons and a glial cell, and the retinal pigmented epithelium (RPE). NR originates from the inner layer of the optic vesicle and RPE from the outer layer, and the separation of the 2 domains is first observed at the early optic vesicle stage. NR and RPE are evolutionally conserved components among animals with eyes, and it is the ideal model system to study the first retinal differentiation. It has been long considered that extracellular secreted molecules from the surrounding tissue of the optic vesicles are crucial for the specification of NR and RPE, but a recent study has shown self-organizing optic-cup morphogenesis in a 3-dimensional culture of ES cells. We focus on a LIM homeodomain-containing gene, Lhx1, which has the ability to transform the prospective RPE to differentiate NR (see figure), to study the molecular mechanisms underlying the induction of NR with the property of retinal stem cells.
References:
- Molecular aspects of eye evolution and development: from the origin of retinal cells to the future of regenerative medicine. Ohuchi H. Acta Med Okayama. 2013 Aug;67(4):203-12. Review.
- Lhx1 in the proximal region of the optic vesicle permits neural retina development in the chicken. Kawaue T, Okamoto M, Matsuyo A, Inoue J, Ueda Y, Tomonari S, Noji S, Ohuchi H. Biol Open. 2012 Nov 15;1(11):1083-93.
3. Analysis of molecular basis for tissue regeneration using an emerging model insect Gryllus bimaculatus
Once we lose a limb, we cannot regenerate the lost part and need to use an artificial limb. However, many animals such as lizards, newts, frogs, small fishes, insects, planaria, and hydra can regenerate lost body parts. Genetic background of regenerative and non-regenerative animals including humans are quite similar, suggesting that no special genes are required for tissue regeneration, but the presence of a difference in reactivation of gene expression after tissue loss. We analyze gene expression during tissue regeneration in an emerging model insect Gryllus bimaculatus to gain knowledge about genetic basis of regeneration. Our final goal is to regenerate the lost body part on the basis of the knowledge obtained from regeneration studies involving Gryllus bimaculatus.
References:
- Leg regeneration is epigenetically regulated by histone H3K27 methylation in the cricket Gryllus bimaculatus. Hamada Y, Bando T, Nakamura T, Ishimaru Y, Mito T, Noji S, Tomioka K, Ohuchi H. Development. 2015 Sep 1;142(17):2916-2927.
- Analysis of RNA-Seq data reveals involvement of the JAK/STAT signalling during leg regeneration of the cricket Gryllus bimaculatus. Bando T, Ishimaru Y, Kida T, Hamada Y, Matsuoka Y, Nakamura T, Ohuchi H, Noji S, Mito T. Development. 2013 Mar; 140(5):959-64.
- Lowfat, a mammalian Lix1 homologue, regulates leg size and growth under the Dachsous/Fat signaling pathway during tissue regeneration. Bando T, Hamada Y, Kurita K, Nakamura T, Mito T, Ohuchi H, Noji S. Dev Dyn. 2011 Jun;240(6):1440-53.
- Regulation of leg size and shape: involvement of the Dachsous-fat signaling pathway. Bando T, Mito T, Nakamura T, Ohuchi H, Noji S. Dev Dyn. 2011 May;240(5):1028-41. Review.
- Regulation of leg size and shape by the Dachsous/Fat signalling pathway during regeneration. Bando T, Mito T, Maeda Y, Nakamura T, Ito F, Watanabe T, Ohuchi H, Noji S. Development. 2009 Jul;136(13):2235-45.
4. Studies on bone formation and remodeling
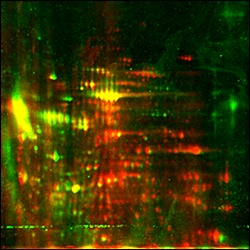
References:
- Effect of risedronate on osteoblast differentiation, expression of receptor activator of NF-κB ligand and apoptosis in mesenchymal stem cells. Fujita H, Kurokawa K, Ogino T, Ono M, Yamamoto M, Oka T, Nakanishi T, Kobayashi N, Tanaka N, Ogawa T, Suzaki E, Utsumi K, Sasaki J. Basic Clin Pharmacol Toxicol. 2011 Aug;109(2):78-84.
- Alpha-tocopheryl succinate induces rapid and reversible phosphatidylserine externalization in histiocytic lymphoma through the caspase-independent pathway. Fujita H, Shiva D, Utsumi T, Ogino T, Ogawa T, Abe K, Yasuda T, Utsumi K, Sasaki J. Mol Cell Biochem. 2010 Jan;333(1-2):137-49.
- Alpha-lipoic acid suppresses 6-hydroxydopamine-induced ROS generation and apoptosis through the stimulation of glutathione synthesis but not by the expression of heme oxygenase-1. Fujita H, Shiosaka M, Ogino T, Okimura Y, Utsumi T, Sato EF, Akagi R, Inoue M, Utsumi K, Sasaki J. Brain Res. 2008 Apr 24;1206:1-12.
- Involvement of Ras/extracellular signal-regulated kinase, but not Akt pathway in risedronate-induced apoptosis of U937 cells and its suppression by cytochalasin B. Fujita H, Utsumi T, Muranaka S, Ogino T, Yano H, Akiyama J, Yasuda T, Utsumi K. Biochem Pharmacol. 2005 Jun 15;69(12):1773-84.